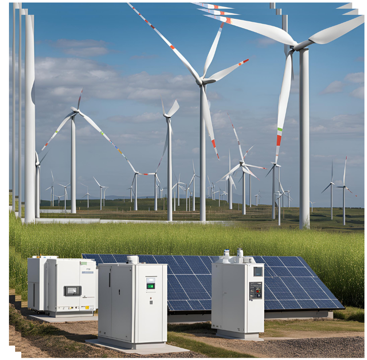
In high-voltage electrical systems, such as gas-insulated switchgear (GIS), circuit breakers, and transmission lines, the choice of insulating gas is critical to ensure safety, reliability, and sustainability. Sulfur hexafluoride (SF6) has long been the industry standard due to its exceptional insulating and arc-quenching properties. However, its significant environmental footprint has spurred the development of alternatives like C4F7N (marketed as Novec 4710 by 3M), a perfluorinated compound introduced as a greener substitute. Deciding between SF6 and C4F7N requires a multifaceted evaluation, weighing economic considerations, environmental impacts, and technical efficiency. Each perspective reveals distinct trade-offs that shape their suitability for modern applications.
Economic Perspective
From an economic standpoint, SF6 has historically been the more cost-effective option. Its widespread use over decades has led to a mature supply chain, with well-established production processes and relatively low per-unit costs. For example, SF6 is produced at scale by chemical manufacturers globally, and its price typically ranges from $10 to $20 per kilogram, depending on market conditions. Additionally, equipment designed for SF6—such as GIS and circuit breakers—has been optimized over time, reducing initial capital costs for utilities and manufacturers accustomed to its specifications.
In contrast, C4F7N is a newer compound, introduced commercially in 2016 by General Electric in partnership with 3M. Its production is less widespread, and the raw material costs are higher due to the complexity of synthesizing perfluoroisobutyronitrile. While exact pricing is proprietary, estimates suggest C4F7N can cost significantly more per kilogram than SF6, often requiring mixtures with buffer gases (e.g., CO2, N2) to achieve practical performance, which adds to handling and blending expenses. Moreover, retrofitting existing SF6-based equipment for C4F7N compatibility or designing new systems incurs additional capital investment. However, long-term economic benefits may favor C4F7N as environmental regulations—like the European Union’s F-gas regulations—impose taxes or penalties on high-GWP gases like SF6, potentially offsetting initial cost disadvantages over time.
Environmental Perspective
Environmentally, the contrast between SF6 and C4F7N is stark, making this a decisive factor for many stakeholders. SF6 is the most potent greenhouse gas known, with a global warming potential (GWP) of 24,300 relative to CO2 over a 100-year period and an atmospheric lifetime of approximately 3,200 years. Even small leaks from electrical equipment—estimated at 1-2% annually—contribute significantly to climate change. In response, jurisdictions like the EU and California have targeted SF6 phase-outs, pushing industries toward alternatives.
C4F7N offers a compelling environmental advantage. Its GWP is approximately 2,750—still higher than CO2 but over 88% lower than SF6—and its atmospheric lifetime is much shorter, around 30 years. When mixed with natural-origin gases (e.g., O2, CO2, N2) at typical molar fractions of 3.5% to 6%, the effective GWP of the mixture drops further, often below 1,000, depending on the blend. This makes C4F7N a viable option for meeting stringent emissions goals. However, as a PFAS compound, concerns linger about its persistence and potential long-term ecological impact, though research on its decomposition products and toxicity remains ongoing. SF6, while also chemically stable, poses no such PFAS-related risks but carries a far heavier climate burden. For organizations prioritizing carbon neutrality, C4F7N is the clearer choice, though neither is entirely free of environmental scrutiny.
Technical Efficiency Perspective
Technically, both gases excel as insulators, but their performance characteristics differ. SF6 is renowned for its unmatched dielectric strength—about 2.5 times that of air—and its ability to extinguish electrical arcs effectively, making it ideal for compact, high-performance switchgear. Its boiling point (-64°C) ensures it remains gaseous under most operating conditions, and its stability allows for decades of use without degradation. These properties have made SF6 the gold standard for high-voltage applications up to 1,200 kV, with equipment designs finely tuned to its behavior.
C4F7N, however, offers a dielectric strength roughly twice that of SF6, enabling even greater insulation efficiency per unit volume. This could theoretically allow for smaller equipment designs, though its higher boiling point (-4.7°C) necessitates mixtures with buffer gases to prevent liquefaction at low temperatures, slightly reducing its effective dielectric strength (typically to 1.5–1.8 times that of SF6 in practical mixtures). Its arc-quenching performance is comparable to SF6’s when properly blended, as demonstrated in commercial products by companies like Hitachi Energy and LS Electric. However, C4F7N’s higher vapor pressure and mixture complexity require more precise engineering and monitoring, increasing technical demands on system design and maintenance. For cutting-edge applications—like the Large Hadron Collider’s high-voltage components—C4F7N has shown promise, but SF6’s proven reliability across a broader range of conditions remains a technical advantage.
Balancing the Trade-Offs
The choice between SF6 and C4F7N hinges on prioritizing these competing factors. Economically, SF6 retains an edge for short-term budgets and existing infrastructure, appealing to utilities with limited capital for upgrades. However, as regulatory pressures mount, C4F7N’s higher upfront costs may be justified by avoiding future penalties and aligning with sustainability mandates. Environmentally, C4F7N is the superior option, offering a dramatic reduction in greenhouse gas impact despite lingering PFAS concerns, while SF6’s climate footprint is increasingly untenable. Technically, SF6’s established performance and simplicity make it a safer bet for reliability, whereas C4F7N’s enhanced insulation potential suits innovative, compact designs willing to tackle its engineering challenges.
For a utility in a region with strict emissions laws (e.g., the EU), C4F7N emerges as the rational choice, balancing environmental compliance with competitive technical performance. Conversely, in areas with lax regulations and budget constraints, SF6 might persist as the pragmatic option until replacements become mandatory. Ultimately, the decision reflects a broader tension between legacy systems and future-proofing—a microcosm of the energy sector’s transition to sustainability.
Conclusion
This essay has explored SF6 and C4F7N through economic, environmental, and technical lenses, revealing no universal “winner” but rather context-specific strengths. SF6 excels in cost and reliability, rooted in decades of use, while C4F7N shines as a forward-looking alternative, driven by environmental urgency and technical promise. Stakeholders must weigh their priorities—whether minimizing immediate costs, meeting climate goals, or optimizing performance—to determine the best path forward in an evolving energy landscape.